DAWN-AF
Digital Twins to Treat Atrial Fibrillation
Atrial Fibrillation (AF) is the most common cardiac arrhythmia, with incidence increasing with age where 10% of the population above 80 years is afflicted with it. As AF is progressive, over time it is harder to treat, which increased risk of stroke, dementia and heart failure. The most effective treatment is catheter ablation therapy (CAT) which selectively destroys tissue to create lesions blocking conduction; however, CAT follows generic patterns, without personalisation. AF often recurs after treatment, with more than 25% of patients requiring re-ablation after 2 years.
We aim to develop a personalised medicine approach based on computer modelling, to plan AF ablation to prevent recurrence. We propose to use physiological digital twins of patient hearts, created from imaging (MRI/CT), and calibrated using machine learning, to analyse and fit ECG and electrogram recordings acquired clinically, from implantable devices or wearables. Novel technology for real-time simulation of AF will be developed and integrated in a clinically viable platform to support the easy flow, robust analysis and interpretation of information, to achieve a scalable translation to large cohorts, and, thus, to enable clinicians to speed up the translation of observations to diagnosis and therapy planning.
Due to inherent uncertainty in measurements, anatomical structures, and properties, multiple AF scenarios will be simulated to derive biomarkers for assessing risk of AF progression, and determine potential ablation sets for each individual prior to CAT. Intraoperatively, electroanatomic recordings will be used on-the-fly to determine which simulation corresponds best to the patient, with its optimal ablation set. Platform development will use large-scale retrospective clinical data, but will be equally applicable to prospective trials. Economic analysis will evaluate benefits arising from early preventative and longer-lasting treatment, reduced duration and procedural risks of interventions.
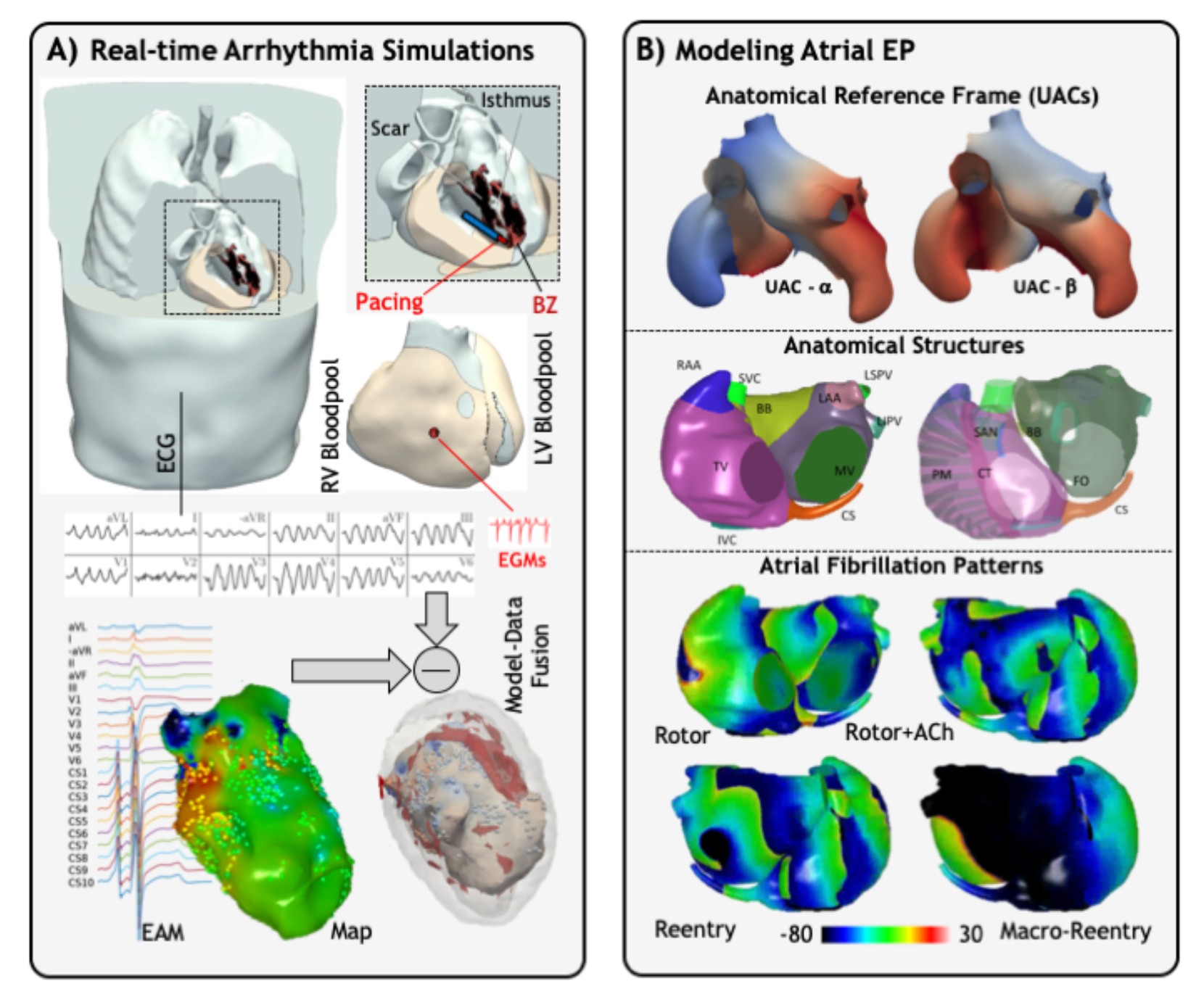
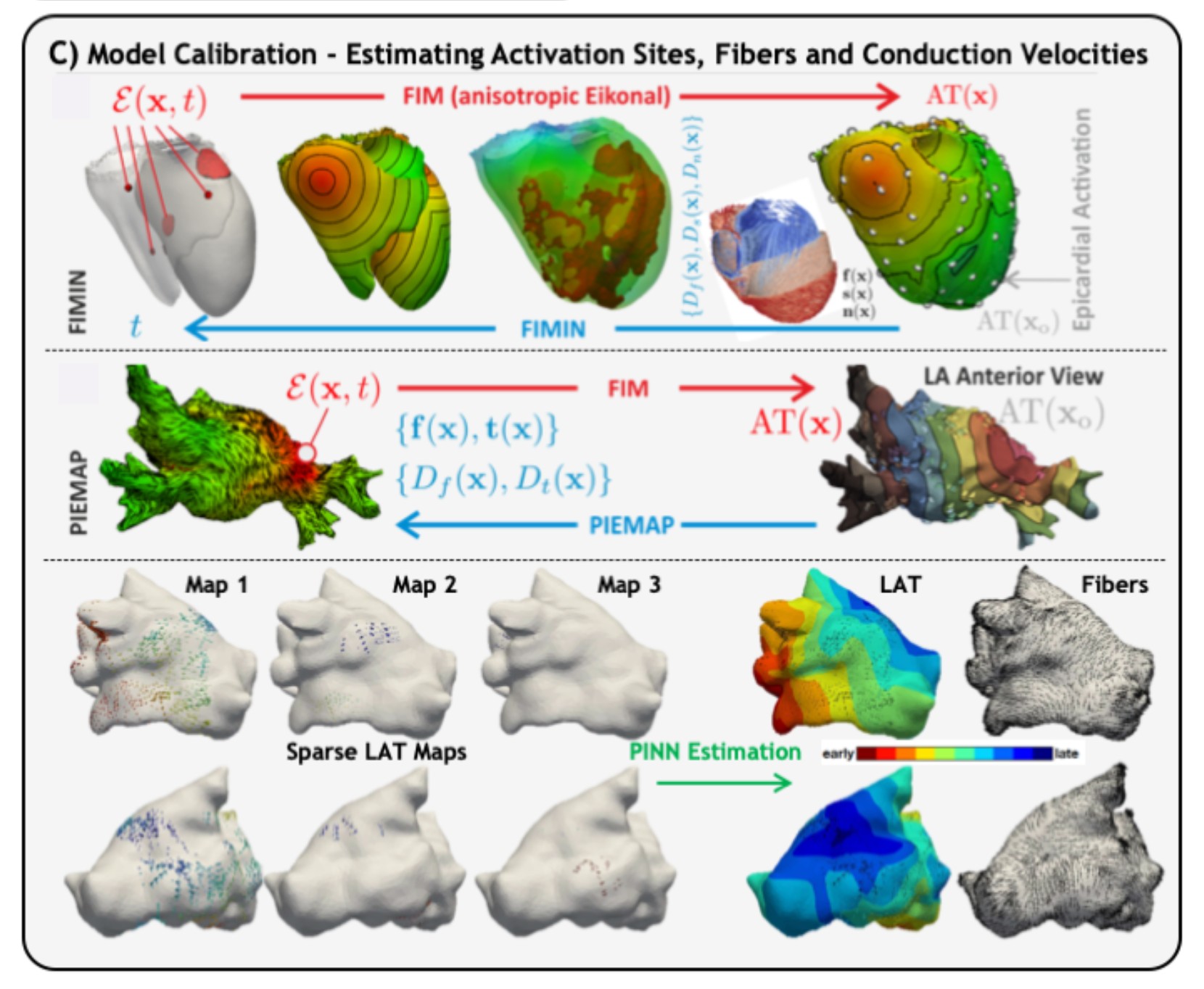
Preliminary results. A) (top) Real-time simulation of ventricular tachycardia entrainment and associated ECG and electrograms; (bottom) co-registration of anatomical model with clinical EAM data. B) (top) Atrial EP modelling work on UACs (24), (middle) detailed representation of anatomical structures, and (bottom) simulations of different AF patterns(28). C) Model calibration techniques for estimating activation sites, fibre architecture and conduction velocities from sparse activation maps for ventricles (top row) and atria (bottom rows) (29). Abbreviations: Electrocardiogram (ECG), Electro-anatomical Mapping (EAM), Border Zone (BZ), Right Ventricle (RV), Left Ventricle (LV), Electrogram (EGM), Electrophysiology (EP), Universal Atrial Coordinates (UACs), Right Atrial Appendage (RAA), Superior Vena Cava (SVC), Bachmann‘s Bundle (BB), Crista Terminalis (CT), Fossa Ovalis (FO), Sino-atrial Node (SAN), Pectinate Muscles (PM), Acetylcholine (ACh), Fast Iterative Method (FIM), FIM-Inverse (FIMIN), Physics-Informed Neural Network (PINN), Activation Time (AT), Local Activation Time (LAT), PIEMAP (23).
Partners: University of Bordeaux, Medical University of Graz, Pontificia Universidad Católica de Chile, Institute for Advanced Studied Austria, University Hospital Center Bordeaux, Alliance du Coeur Sudouest France
We currently have a postdoctoral position opening for this project. More details are found here.
HELP
Unique non-invasive pace-mapping system to identify subjects at risk of arrhythmic sudden death
Sudden cardiac death (SCD) is a common cause of adult mortality in western countries, accounting in Europe for about 350 000 cases annually. Most SCDs are caused by ventricular arrhythmias generated from an arrhythmogenic ‘substrate’ present within the heart. Paradoxically, despite the existence of efficient preventive therapies, the sole available predictor of SCD is a measure of cardiac contractility, an indirect metric, which applies only to a subset of patients. At present, most patients at risk cannot be identified pre-emptively to prevent sudden death.
The aim of this project is to develop a novel non-invasive body-surface mapping and pacing system, which will allow detection of cardiac signals related directly to the substrate responsible for lethal arrhythmias, for efficient SCD prediction.
The unique approach proposed to achieve this objective will consist in: (1) combining electrocardiographic mapping and ultrasonic pacing technologies during cardiac signal acquisition from a high-density array of body surface electrodes; (2) characterizing micro- scale temporal, spectral and spatial features of substrate signals, at baseline and during pacing to unmask hidden signals; (3) establishing critical signal features specific of arrhythmogenic substrates using multi-parametric signal analysis on the body surface, based on unique electrophysiological data from explanted human hearts and from SCD survivors; (4) developing risk prediction scores from well-phenotyped groups of patients monitored by implanted devices.
This project will constitute a new paradigm in clinical cardiac investigations and allow a major breakthrough in the prevention of premature arrhythmic deaths in the world. The capability of detecting and influencing cardiac electrical signals will also dramatically impact the management of populations suffering from other cardiac pathologies, enabling earlier diagnosis of heart disease, and better guidance to drug, interventional or preventive therapies.
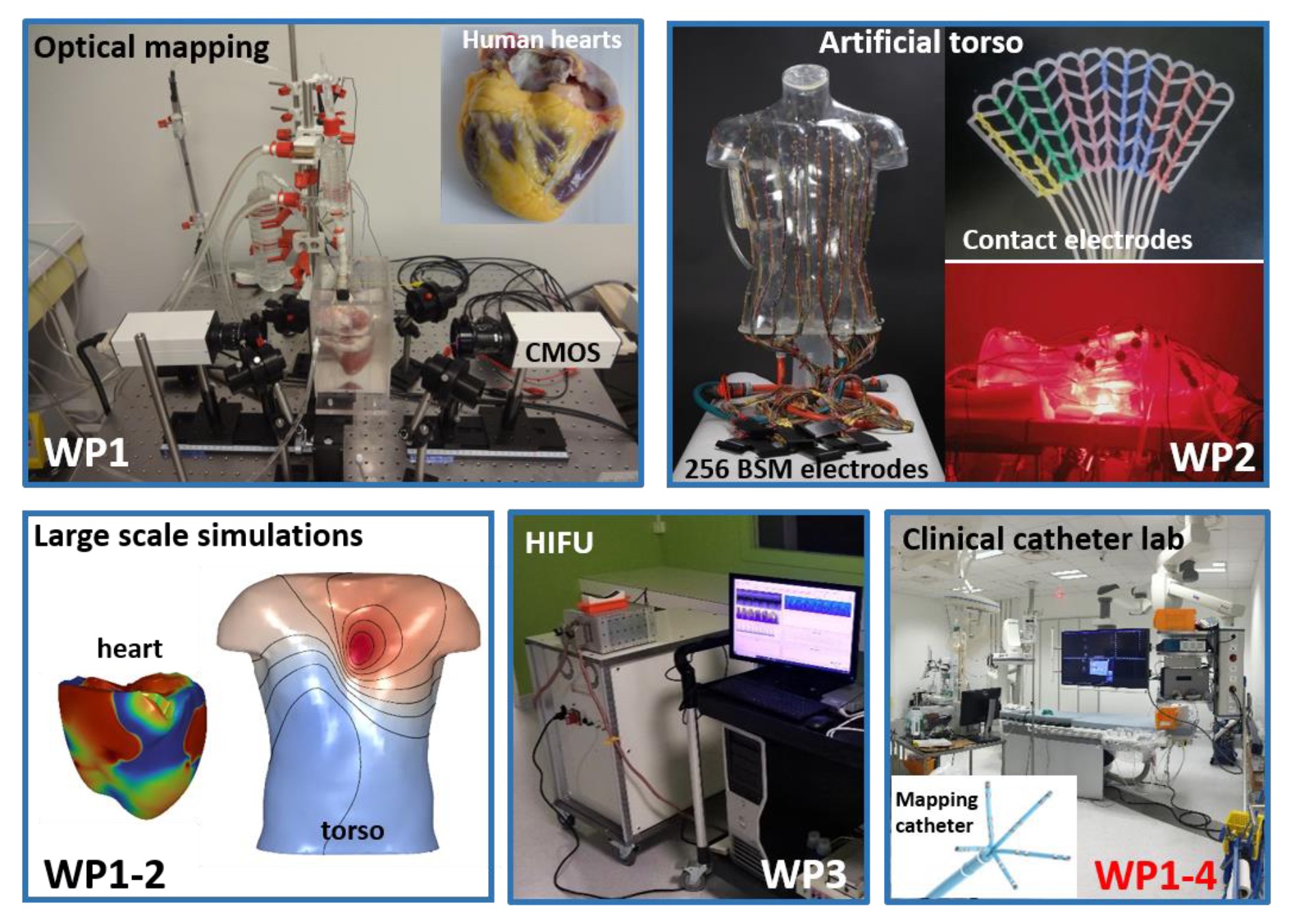
Participants: University of Bordeaux
V-SMART
Ventricular Scar-Mediated Arrhythmogenesis
Cardiac disease is the number one cause of death in the industrialized world. Ischaemic episodes, commonly referred to as heart attacks, affect more than 60,000 people in France per year (500,000 in Europe), resulting in 14% of all deaths. Women currently account for about 1/3 of all cases, but this will increase as the average age of women experiencing myocardial infarction (MI) is decreasing. There is an acute phase during which the risk of arrhythmia is very high. As time progresses, necrosis and apoptosis occur, creating a MI from the replacement of myocytes by fibrosis, forming a scar. It is well recognized that post-MI patients are at increased risk for ventricular arrhythmias, one which does not diminish over time as scars are permanent and myocytes do not regenerate. As a consequence, many patients receive prophelactic implantable cardioverter defibrillators (ICDs) to terminate arrhythmias via electric shock.
Several well known factors contribute to the arrhythmogenicity of the post-MI heart. The scar architecture is complicated and may have narrow, slowly conducting channels which allow for the easier establishment of reentrant loops. Furthermore, the area immediately adjacent to the scar, called the border zone, has different electrophysiological (EP) properties. Alterations in the ionic currents and calcium handling properties lead to a shortened action potential duration (APD), making reentry easier. While conducting channels within scar leading to monomorphic ventricular tachycardia (VT) are well understood, other mechanisms contributing to ectopic beat formation, and polymorphic VTs or fibrillation remain unelucidated. An aspect not well researched is the electromechanical (EM) interaction at the scar border. Scars do not contract and are are stiffer. This will lead to increased stress at the border of the scar, and could result in significant MechanoElectric Feedback (MEF) effects, which are known to be strong enough to dramatically affect electrophysiology (EP), inducing arrhythmia. Measuring EM activity in vivo is very difficult, especially at the resolution required in the setting of scar. CINE MRI records motion but resolution is coarse, and strain, besides also being coarse, is not measured along myocyte orientation. Explanting hearts changes pressure and pericardial boundary conditions, leading to a very different contraction. Trying to capture electrics and mechanics simultaneously is even more problematic in a beating heart. Modelling is currently the best paradigm for disentangling mechanisms when studying organ level EM interaction as data at all scales in the model are known and available, including structural (tissue fibre orientation) and EP, at high spatiotemporal resolution.
We hypothesize that mechanical consequences of cardiac scar, in addition to electrophysiological remodelling, strongly increase arrhythmogenic risk. To demonstrate this, a combination of biological experiments and computer simulations will be performed. The biological experiments will confirm the hypothesis, and provide functional data for MEF parameterization. With this new physiological knowledge, personalized patient EM heart models with infarcts at an unprecedented resolution will be created, and potential therapies investigated in silico.
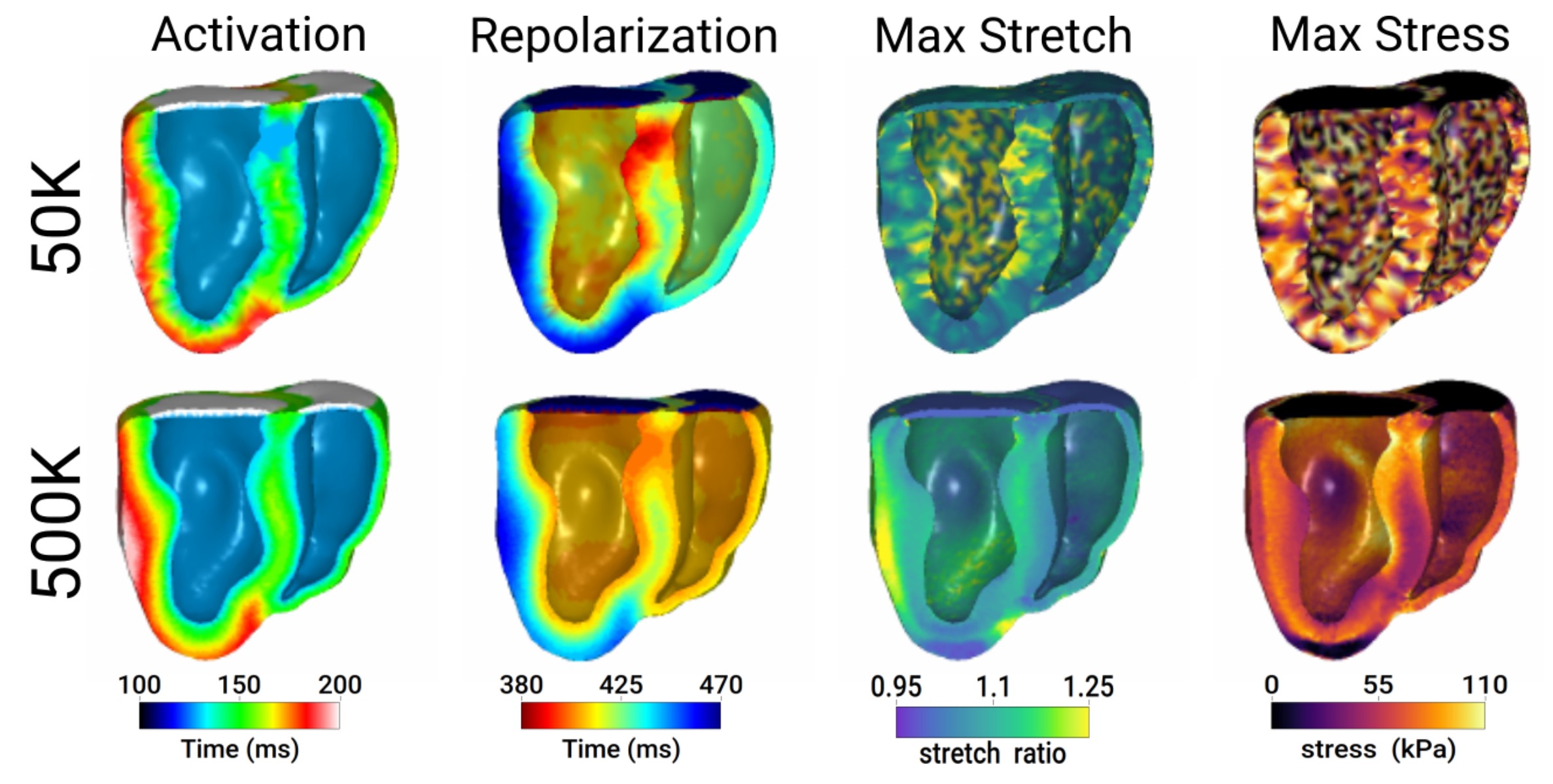
Effect of model discretization on electromechanical simulations. A dog heart was discretized into 50k (top row) or 500k (bottom row) tetrahedral elements. From left to right: Electrical activation time, repolarization time, maximum stretch, and maximum stress developed.
Partners: University of Bordeaux: Liryc, IMB, INSERM, CHU
SICVALVES
Multiscale modeling of Valvular Heart Diseases. Understanding the mechanisms of adverse remodeling to improve precision medicine
Valvular Heart Diseases (VHD) are chronic-rogressive diseases that vary with gender and age. If left untreated, VHDs can cause malignant arrhythmias and severe heart failure. The existing guidelines for treatment are using only rough function parameters that fail to account for inter-individual variability. Nowadays, computational modeling has the potential to unveil important pathophysiological mechanisms and to contribute towards personalized precision medicine. The objective of this project is to use advanced models of the cell and tissue up to the organ level in VHD to gain mechanistic insights about triggers of ventricular arrhythmias, diastolic, and systolic dysfunction and myocardial metabolic alterations and how these processes reinforce each other. Gender differences will be systematically taken into account, to improve diagnostics, risk assessment, and treatment planning of VHDs. Existing models of biomechanics, electrophysiology, and hemodynamics will be optimized for individual cases using a pool of data from our own previous trials. Model parameters will be calibrated under pre- treatment conditions and models will be validated by comparing model predictions of acute post- treatment conditions against clinical data. Finally, longer term follow up data of cases will be used to enable predictions of chronic outcomes.
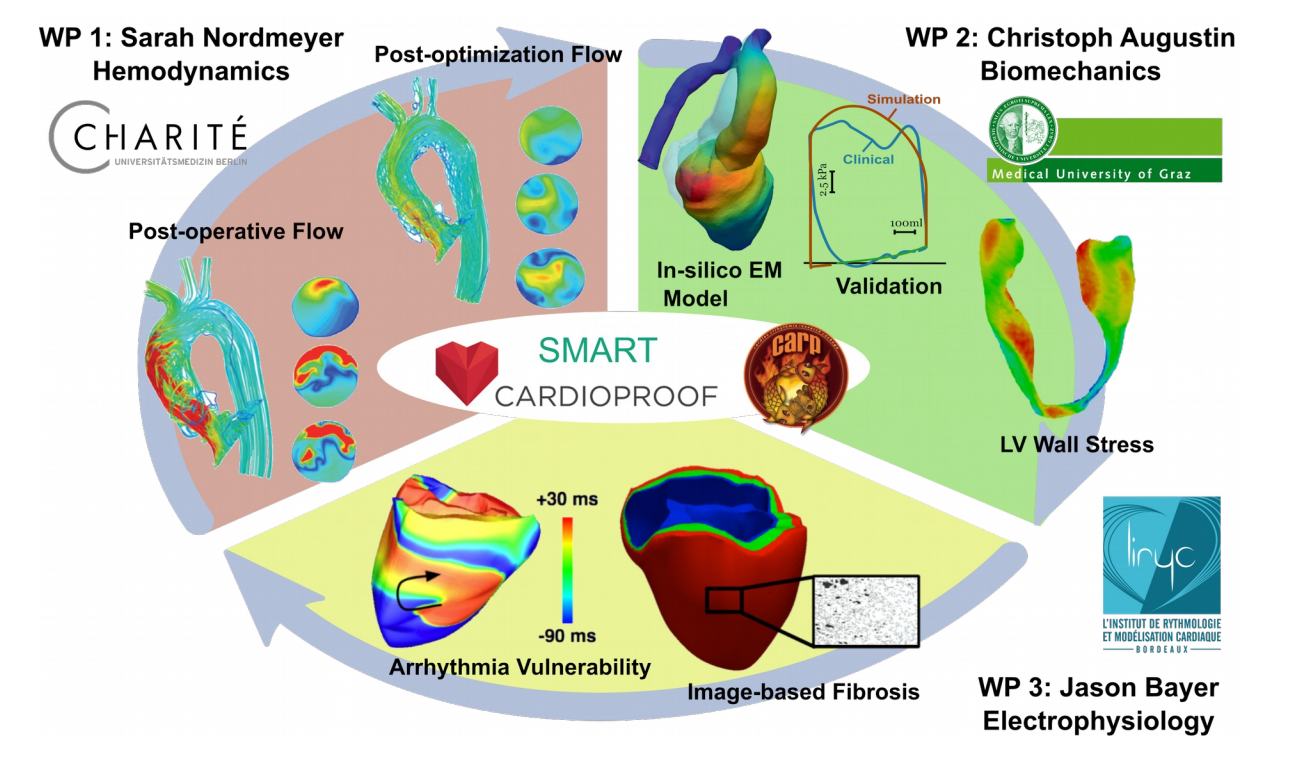
Preliminary simulation results showing: WP 1 - Optimization of postoperative hemodynamics; WP2 - Clinically validated EM models of LV and aorta. Wall stresses, which can only be computed using in-silico methods, play a pivotal role in adaptation processes in AS patients; and WP 3 - the ability to generate arrhythmias in the human ventricles with image-based fibrosis. Rich clinical datasets are made available from the EU FP7 Cardioproof and SMART projects, and the numerical framework is implemented in the modeling software CARPentry.
Partners: University of Bordeaux, Medical University of Graz and Charité - Universitätsmedizin Berlin
ATLAS-RVA
Atlas of normal and pathological ventricular Repolarization patterns and correlation with clinical re-entrant Ventricular Arrhythmias
The role of repolarization in the onset and maintenance of re-entrant rhythms that give rise to ventricular tachycardia (VT) is not clear. Already, what constitute typical repolarization time (RT) patterns in the ventricles under sinus rhythm is disputed. The first goal of this project is to provide detailed insight into such patterns. This will be done primarily via RT annotations in (appropriately filtered) electrograms from in-vivo human patient datasets, but the project also considers new and alternative methods of RT annotation. The datasets form a source of in-vivo data under sinus rhythm for performing such a study, and also mappings of re-entrant circuits. Hence, we also propose to develop numerical models that are capable of mimicking VT-patient physiology in order to further investigate how such RT patterns modulate the vulnerability of tissue to re-entrant circuits. The success of this project will lead to a valuable reference in cardiac electrophysiology by way of a statistically supported atlas of ventricular repolarization that documents typical patterns under physiological conditions and how such patterns may vary. While the focus at this stage is on dispersion of repolarization, the VT models will also permit more detailed study into other factors such as the role of tissue restitution and assessing treatment strategies.
Partners: Peter Langfield (INRIA IHU Liryc) & Karim Benali (L'hôpital Haut-Lévêque)